What Does a Sun-Like Star
Eventually Become?
- Planetary nebula: He shell flashes
and ejection of the star's envelope, ionized by the star's UV
radiation; May look round (Ring Nebula or NGC 3132) but most
have bipolar lobes (NGC 2346 or Henize 3-401), probably because
of magnetic fields, and possibly due to orbiting companions;
[The closest known in the Helix nebula, 450 ly away].
- Dying star: By this time, it
has shed almost half its mass, and no more matter remains around
it either, including nearby planets it may have had; Distant
ones may survive, but they become warmer and ice on their moons
or "Kuiper belt" objects may turn to gas.
- White dwarf: From the hot remnant
of the C core, cooling down; Earth-sized but with the Sun's mass,
stabilized by electron degeneracy pressure.
- Examples: The first one discovered
was Sirius B (small but very hot); We know other ones, but they
are hard to see unless they are in binary systems...
|
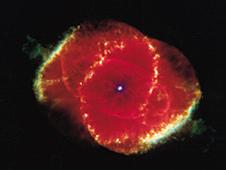
- End of evolution: The star becomes a
black dwarf (unless more matter falls on it), some of its matter is
added to the surrounding interstellar medium.
|